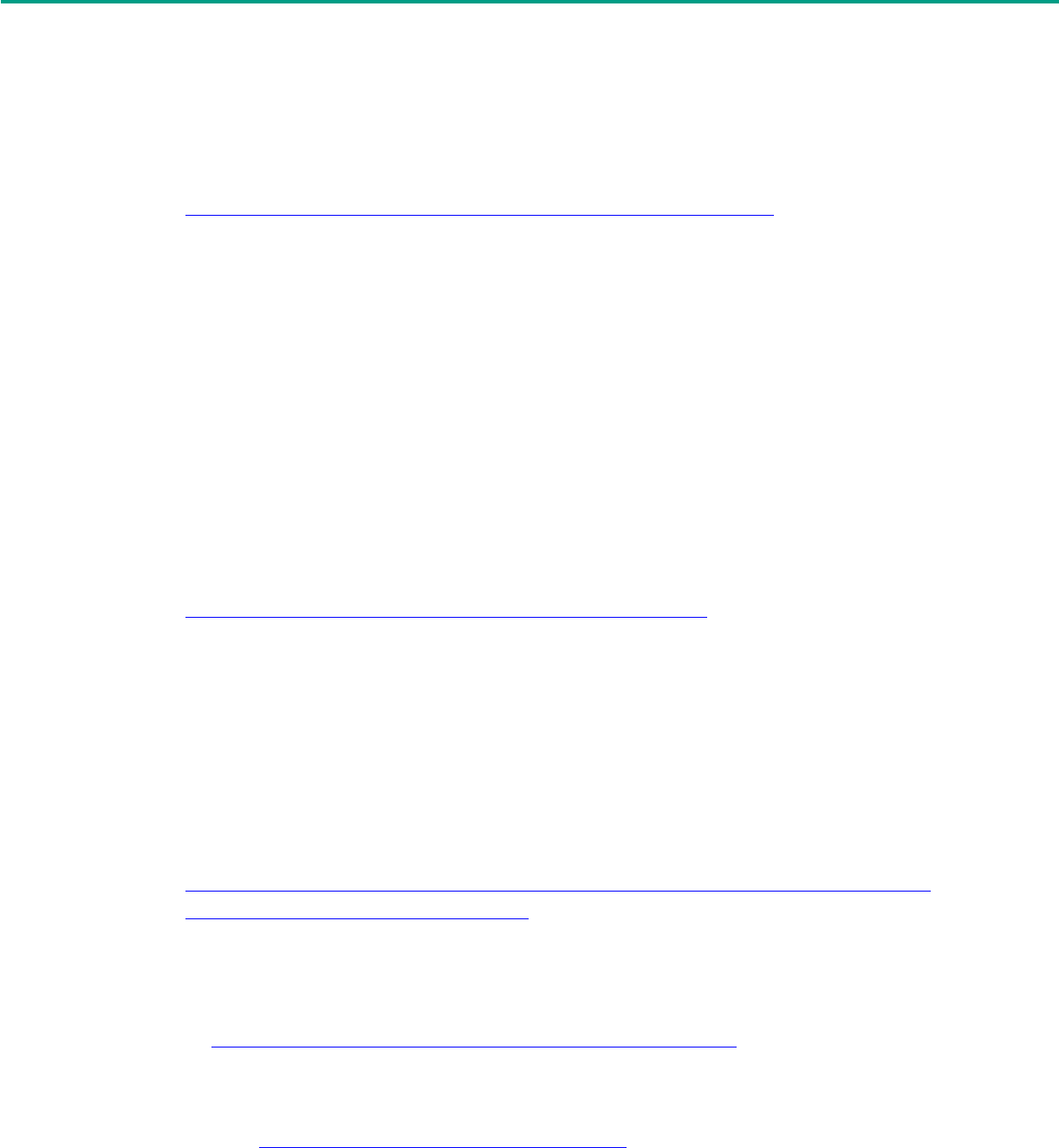
HIGHWAY SAFETY BENEFIT COST ANALYSIS GUIDE REFERENCES
143
REFERENCES
1. Sallman, D., E. Flanigan, K. Jeannotte, C. Hedden, and D. Morallos, Operations Benefit/Cost
Analysis Desk Reference, FHWA-HOP-12-028, Federal Highway Administration,
Washington, DC, 2012. Accessed February 2018 at:
http://ops.fhwa.dot.gov/publications/fhwahop12028/fhwahop12028.pdf.
2. Srinivasan, R., F. Gross, B. Lan, and G. Bahar. Reliability of Safety Management Methods:
Network Screening, FHWA-SA-16-037, Federal Highway Administration, Washington,
DC, 2016.
3. Srinivasan, R., G. Bahar, and F. Gross. Reliability of Safety Management Methods: Diagnosis,
Report No. FHWA-SA-16-038, Federal Highway Administration, Washington, D.C.,
November 2016.
4. Bahar, G., R. Srinivasan, and F. Gross. Reliability of Safety Management Methods:
Countermeasure Selection, Report No. FHWA-SA-16-039, Federal Highway
Administration, Washington, D.C., August 2016.
5. Code of Federal Regulations, Highway Safety Improvement Program, 23 CFR 924.3,
Accessed February 2018 at:
https://www.fhwa.dot.gov/legsregs/directives/fapg/cfr0924.htm.
6. Gross, F., T. Harmon, G. Bahar, and K. Peach. Reliability of Safety Management Methods:
Systemic Safety Programs, FHWA-SA-16-041, Federal Highway Administration,
Washington, DC, 2016.
7. American Association of State Highway and Transportation Officials (AASHTO),
Highway Safety Manual, 1
st
Edition, 2010.
8. US Department of Transportation, Economic Analysis Primer, FHWA-IF-03-032, Federal
Highway Administration, Washington, DC, 2003. Accessed February 2018 at:
http://www.webpages.uidaho.edu/~mlowry/Teaching/EngineeringEconomy/Supplemental/
USDOT_Economic_Analysis_Primer.pdf.
9. Lawrence, M., P. Nguyen, J. Skolnick, J. Symoun, J. Hunt, and R. Alfelor. Transportation
Systems Management and Operations Benefit-Cost Analysis Compendium, FHWA-HOP-14-
032, Federal Highway Administration, Washington, DC, 2015. Accessed February 2018
at: https://ops.fhwa.dot.gov/publications/fhwahop14032/index.htm.
10. Harmon, T., G. Bahar, and F. Gross. Crash Costs for Highway Safety Analysis, FHWA-SA-
17-071, Federal Highway Administration, Washington, DC, 2018. Accessed February
2018 at: https://safety.fhwa.dot.gov/hsip/planning.cfm.